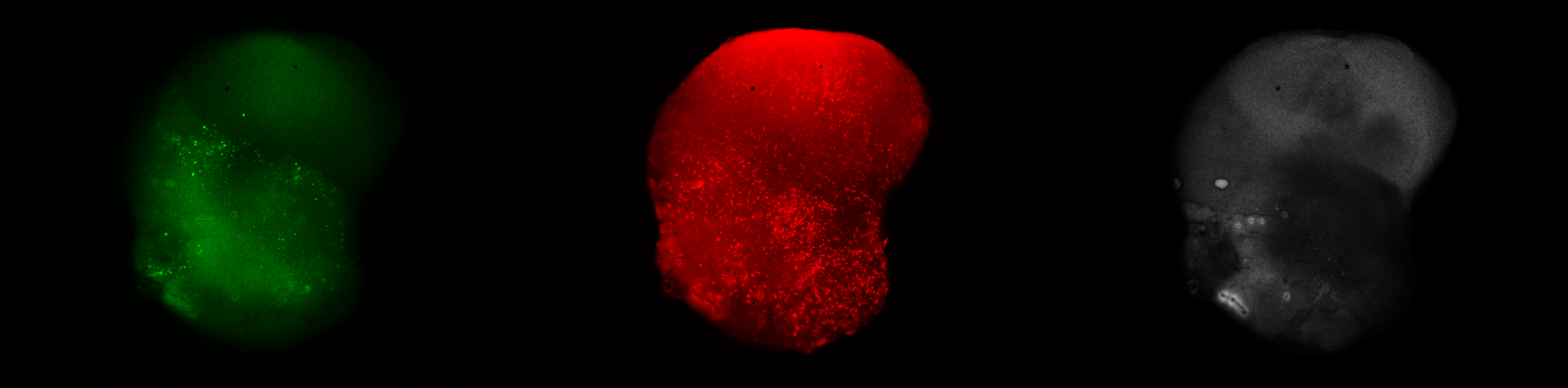
Research in the Lybrand lab
3D Models of TBI Neural Network Dysfunction after Traumatic Brain Injury
Traumatic brain injury (TBI), particularly from blast exposure, can profoundly disrupt how neurons communicate — not just at the cellular level, but across entire networks. In the Lybrand Lab, we study how these injuries impair large-scale neural synchrony and circuit organization, leading to cognitive and behavioral dysfunction.
Our work focuses on identifying how networks transition from healthy, highly organized states into dysfunctional or “fragile” configurations after trauma. Using high-resolution electrophysiology, we record from hundreds of neurons simultaneously in brain slice cultures and organoid systems. We then apply graph theory and custom network modeling to track shifts in connectivity patterns — including changes in synchrony, clustering, and hub structure.
We’ve found that after blast injury, networks often fragment or lose central nodes that are critical for efficient information flow. These changes are dynamic, sometimes transient, and can provide early markers of long-term dysfunction. By characterizing these patterns, we aim to uncover the principles of network failure and recovery, ultimately informing the development of targeted interventions.
This research is part of our broader effort to bridge cellular pathology with systems-level dysfunction — and to develop biomarkers and therapeutic strategies that address the full complexity of brain injury.
Explore our open-source tools for network dynamics
Modeling bTBI-induced hearing loss with otic organoids
Hearing loss is one of the most common and often overlooked effects of blast exposure, frequently co-occurring with traumatic brain injury. In the Lybrand Lab, we are building human otic organoids — 3D inner ear models derived from pluripotent stem cells — to investigate how blast overpressure damages the sensory hair cells of the cochlea.
These models allow us to recapitulate the developmental stages of the inner ear and identify how mechanical injury disrupts hair cell development and survival. Our goal is to uncover human-specific mechanisms of damage and regeneration in the auditory system, with an eye toward developing therapies that restore hearing after trauma.
We combine stem cell biology, advanced imaging, transcriptomics, and functional assays to bridge cellular damage to sensory dysfunction. This work supports our broader mission of understanding how blast trauma affects both the brain and sensory systems.
This work is supported by the Department of Defense
Cortical Grafts for Repair After Traumatic Brain Injury
Restoring function after traumatic brain injury (TBI) remains one of the greatest challenges in neuroscience. Our lab is developing human iPSC-derived cortical grafts to promote recovery after injury to the motor cortex. These 3D cortical structures are composed of region-specific excitatory and inhibitory neurons, mimicking the layered architecture of the human cortex.
We transplant these grafts into mouse models of TBI to evaluate how different cell compositions influence motor recovery and functional integration with the host brain. Using behavioral testing, viral tracing, and histological analysis, we track both recovery of motor function and the formation of synaptic connections between grafts and host neurons.
Our long-term goal is to define the cellular and circuit-level features that make a graft therapeutically effective. This work supports our broader mission to develop regenerative strategies for brain repair — bridging stem cell biology, neural engineering, and functional recovery after trauma.